Saving Lives: CFD Simulation of a Mechanical Heart Valve
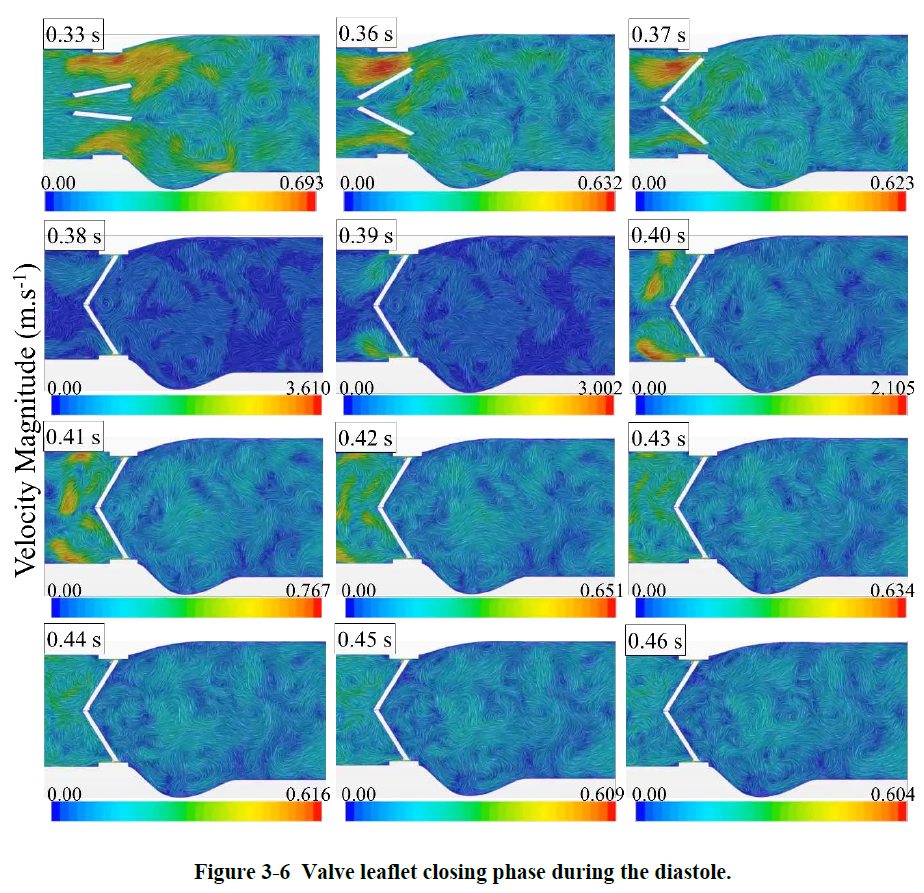
Kristian: Hi Fardin, and congratulations on finishing your Ph.D. Simulation and Medical Device Design, a topic after my taste. As a Mechanical Engineer, what motivated you to move into this field and why did you choose this topic for your thesis?
Dr Fardin KhaliliFardin: Hi Kristian. Thank you. I received my Ph.D. in Mechanical Engineering from the University of Central Florida.
As a student of Mechanical Engineering, I have always had a passion for CFD. In 2012, I started working on flow analysis over a cylinder using Simcenter STAR-CCM+ to investigate the turbulence and flow separation over bluff bodies.
I then completed a Master’s Thesis working on CFD and aeroacoustics analyses of a horizontal wind turbine. Moving to CFD of internal flows especially for medical applications, users may face different kinds of challenges regarding the accuracy of their CAD model, boundary conditions, material properties, fluid-structure interactions (FSI), etc. My Ph.D. research work was about CFD and sound analysis of a bileaflet mechanical heart valve.
Kristian: What was your motivation for this work?
Fardin: Cardiovascular diseases (CVDs) are the leading causes of death in the world. Hence, understanding of normal cardiac functions and diseases is vital for diagnostics and treatments. Computational fluid dynamics (CFD) and finite element analysis (FEA) have the potential to explain and enhance clinical observations and provide detailed information of the blood flow and its interactions with blood vessel and tissue layers. The detailed information that can be provided by CFD and FEA might not be accessible with in-vivo measurements.
Kristian: So, it is the technical challenge and fascination with CFD and FEA, as well as the desire to drive innovation in medical device design, right?
Fardin: Absolutely: I believe that if someone is expert in the accurate modeling and fluid dynamics analysis of biological systems with all the challenges from setting up the initial and boundary conditions to applying moving mesh and FSI methods, then, they can run perfect simulations for applications in all other industries.
Kristian: I agree. So for your dissertation, you worked on a bileaflet mechanical heart valve model, right? Can you tell us more about this?
Fardin: The heart valve geometry was modeled based on St. Jude Regent Medical heart valve. In the illustration, the BMHV is in the fully open position and divides the flow into three orifices: two of them (the top and bottom orifices) are roughly semicircular, and the third (middle orifice) is almost rectangular. In addition, we used a realistic geometry of the aortic sinuses, since it is important for appropriate flow field analysis. Dynamic Fluid Body Interaction (DFBI) and overset mesh features were used to simulate the motion of multiple objects with different meshes that overlap each other. It is important to note that the DFBI Six-DOF solver was frozen for the initial two cycles. Then, the solver was activated; however, a motion limit was assigned to the leaflets to prevent a sudden large displacement and a consequent solution divergence. This approach also helps the flow to reach its periodicity.
(a) 3D model of a bileaflet mechanical heart valve; (b) Front view of aortic root sinuses; (c) Prospective; and (d) cross-sectional views of the overset mesh and flow domain
Mechanical heart valves rely on reverse flow to close their leaflets. You can find videos of velocity distributions in valve leaflet opening and closing phases during one cardiac cycle on my LinkedIn profile (https://www.linkedin.com/feed/update/urn:li:activity:6378579201003442176, and https://www.linkedin.com/feed/update/urn:li:activity:6394588650172223488).
The blood flow exerts forces on the two leaflets in the acceleration period time until they reached their fully open position at ~0.06 s of the cardiac cycle. In the deceleration period small vortical structures emerge with high fluctuations downstream of the valve. During the diastole, the blood flows back through the valve as the leaflets are closing. The leakage can also occur when the leaflets are fully closed from the gap between the leaflets and valve housing. It is interesting that the backflow velocity raised up to about 3.6 m/s along with high fluctuations which are higher that the velocity magnitude of 2.1 m/s at the peak systole. Some information on how these fluctuations can increase the risk of blood damage and platelet activation can also be found in this link: https://www.linkedin.com/feed/update/urn:li:activity:6389912348227878912).
Valve opening
Valve closing
Kristian: Great, thank you for that explanation. You mentioned ‘small vertical structures’ and ‘high fluctuation’ which triggers a question that often comes up: Can you tell us generally about the role of turbulence in these biological flows?
Fardin: Research studies on low Reynolds number flows are less common, although they are highly relevant to biomedical applications. These flows with such low Reynolds number are considered turbulent include those created through glottis in the upper airways and blood flow through the aortic heart valve. Solving the flow fluctuations accurately leads to better understanding of sound propagations from biological systems. I performed an experiment to study the airflow through a constricted pipe and the suitability of different turbulence models. I ran the simulations in Simcenter STAR-CCM+ and validated the results using Laser Doppler Anemometry (LDA) velocity measurements. Analyses of the mean velocity and local velocity fluctuations indicated that the LES Smagorinsky subgrid-scale turbulence model had the highest agreement with experimental results.
Kristian: How do you think these heart valves can be further improved? Can you tell us about the limitations they have today and where do you think we will be with this technology in 5 and 10 years?
Fardin: Prosthetic heart valves have gone through many design enhancements over about 70 years. To achieve an ideal hemodynamic performance of prosthetic heart valves, there is still a need for design improvements for reducing the risk of potential blood damage, platelet activation, regurgitation and other issues. On the other hand, for further improvements of such medical devices there should be a common interest between engineers and clinicians to increase their cooperation. Clinicians are sometimes more interested in sounds and signals from biological systems such as heart signals and sounds from lung airways. I think “sound” would the bridge between these two groups of people. CFD and FEA are capable of supporting sound studies too.
Kristian: And how about limitations on the simulation side? Do you believe simulation is ready to support clinical trials for devices like heart valves?
Fardin: Approximation is inevitable in computational simulations, but how much they are accurate and reliable is a big deal. Certainly, verification and validation of the methods used in any study must be the first steps. CFD simulations have already had remarkable impacts on many different fields and industries. There are many computational studies worked on CFD analysis of biological systems (such as lung airways, heart valves and left ventricle, aneurysms, etc.), which have provided useful information to at least explain the clinical observations and allow immediate feedback for actual clinical experiences. There are still many unknowns including the accurate material properties, boundary conditions, and physiological interactivities. The cooperation between engineers and clinicians will, hopefully soon, further make simulations ready to support clinical trials. It is also good to note that some criteria such as the number of mesh cells and very low time steps for such simulations make it hard for students for having an accurate simulation setup and run it on their computers.
Kristian: What are your next career steps? Where do you believe you can contribute best from what you learned during your PhD?
Fardin:
My studies have provided me with solid knowledge in three-dimensional CAD solid modeling and physics-based simulation tools. Throughout my graduate studies, I provided advanced engineering analysis and numerical modeling for highly diverse fluidic mechanical and biomedical applications utilizing computational fluid dynamics (CFD) and finite element analysis (FEA). In addition, the privilege of supervising several experimental projects, including hands-on experiences in developing experimental setups, prototyping, and instrument testing for validation purposes, has also given me the understanding of leadership and project management along with proficiency in vast range of software skills.
My role model is Christopher Penny who is working at Siemens and has climbed the ladder of success from an Application Engineer Support to Academic manager in the Americas. In 2013, I opened a case on Steve Portal, and he, as an Application Engineer Support at that time, created a video to teach me, in detail, how to step-by-step fix and run my simulation, a great way that made me interested in CFD and familiarized me with many features in STAR-CCM+. I believe I can have remarkable positive impacts on people as well. I would like to tackle developments of algorithms/methods that will enable the application of the latest CFD/FEA techniques and experimental fluid science to industrial-scale problems.
Kristian: Fantastic. Thank you Fardin for sharing this material with us. And all the best for your future endeavors.
Fardin: I would like to thank you for giving me this opportunity to talk about my research. I believe recognizing and respecting others’ efforts will make them motivated and eager to study, learn, and work with passion.
PS: Stay tuned for the upcoming webinar with Fardin to learn even more about his work.